RP 2: High-resolution imaging of Ca2+ dynamics of microdomains in excitable cells using chemical dyes
Ca2+ signaling is essential for all cells of the organism, where it controls many metabolic reactions. However, it is especially relevant in neurons and cardiomyocytes, where it regulates functional reactions from the millisecond time scale, as in the case of synaptic release and cellular contraction, to long-term changes, including synaptic plasticity or switches in gene expression patterns. Ca2+ signals have been investigated for several decades in these cell types, but our knowledge is still severely limited, especially on how cells generate and distinguish distinct Ca2+ signals. Moreover, we do not understand how Ca2+ dynamics behave in cellular sub-compartments and even less in microdomains. This is due to the fact that the fluorescent sensor molecules currently used to reveal Ca2+ signals do not allow us to obtain such information. Two main types of sensors are currently employed: 1) chemical dyes which are allowed to penetrate into the cells, where they become trapped in the cytosol, which enables them to report cytosolic Ca2+ dynamics and 2) fluorescent protein chimeras that are encoded genetically in the cells, where they can be targeted to the cytosol or to specific organelles, such as endoplasmic reticulum or mitochondria, to report the respective Ca2+ dynamics. Both types of sensors have severe limitations. Genetically encoded Ca2+ sensors suffer from narrow dynamic ranges and slow response times, while chemical dyes are very difficult to target to specific sites in the cell. These limitations severely affect our current understanding of Ca2+ signals.
To solve this, we propose to combine the advantages of chemical dyes and genetically encoded sensors by genetical targeting of chemical dyes. This will enable us to understand rapid dynamics in specific microdomains, taking advantage of the high Ca2+ sensitivity, light throughput, signal-to-noise ratio, response time and dynamic range of chemical dyes. Our approach will improve fundamentally both the temporal and the spatial resolution of Ca2+ imaging in neurons and cardiomyocytes. This work will be enabled by combining the expertise in cell imaging and genetic manipulation of the Brügmann lab with the protein tagging and nanoscale labelling expertise of the Rizzoli lab. We propose to employ three major complementary tagging strategies. First, we will rely on self-labelling enzymatic tags such as the Halo- or SNAP-tag, which allow the direct covalent binding of the fluorescent dye to the protein of interest, and which we have already used in live cells. Second, we will rely on epitope tags such as the FLAG or Strep tags, or the recently developed ALFA tag, which can be targeted by nanobodies or intrabodies. Third, we will rely on click chemistry, which we have optimized for cellular imaging for several years. Importantly, these targeting strategies can be applied both to intracellular compartments and to extracellular sites as the synaptic cleft or the t-tubules of cardiomyocytes.
For an initial trial of experimental conditions, we collaborated closely with the group of Kai Johnsson (Department of Chemical Biology, MPI Heidelberg), who provided us with a Silicon rhodamine MaP Ca2+ indicator (Sir-MaP Ca2+) dye, which can be excited in the far-red range (with 650 nm) and shows a 6-fold increase in light emission upon maximal Ca2+ saturation. Importantly, Sir-MaP Ca2+ binds covalently to the protein of interest via a HaloTag. Thus, we explored the feasibility of Ca2+ imaging within HEK293 cells expressing the HaloTag protein targeted to the mitochondrial matrix with the MTS targeting sequence. Dual imaging in combination with cytosolic Fluo4 dye allowed us to quantify in detail the kinetics of Ca2+ diffusion from the cytosol into the mitochondrial matrix after stimulation with ATP. We excluded any cross-contamination between both signals by comparing the maximum increases and the kinetics in cells loaded with single dyes, in comparison to those loaded with both dyes. Thus, we can demonstrate selective targeting of compartments and the possibility of multiplexing for exact quantification of individual Ca2+ dynamics, which will be later extended to specific microdomains in cardiomyocytes and neurons. Importantly, using a far-red shifted Ca2+ dye allows multiplexing with Ca2+ imaging of other microdomains, as well as simultaneous imaging of other second messengers and signaling effectors.
Our work has a very strong innovation potential, since our approach could be employed with many other types of sensors, to record changes in other ions and second messengers, or to analyze ROS production. Importantly, the same transgenic cells and animals expressing certain tags can then be used for different imaging in parallel experiments, enabling us to determine the exact dynamics and kinetics between the different signaling effectors in this specific microdomain.
Overall, the impact of our work is two-fold. First, we will generate a technology that will enable a more precise and detailed analysis of both neurons and cardiomyocytes, and will allow an analysis of the fundamental similarities and differences in their physiology, in a fashion that was not possible in the past. Second, we generate here a platform that will enable us to teach, at the graduate level, a collection of technologies, from live imaging to super-resolution, genetics, probe development and optogenetics, which is, to our knowledge, unique in Germany, and therefore especially suitable for the present application. We exemplify this in the following paragraphs, in which we explain the work to be performed by the students.
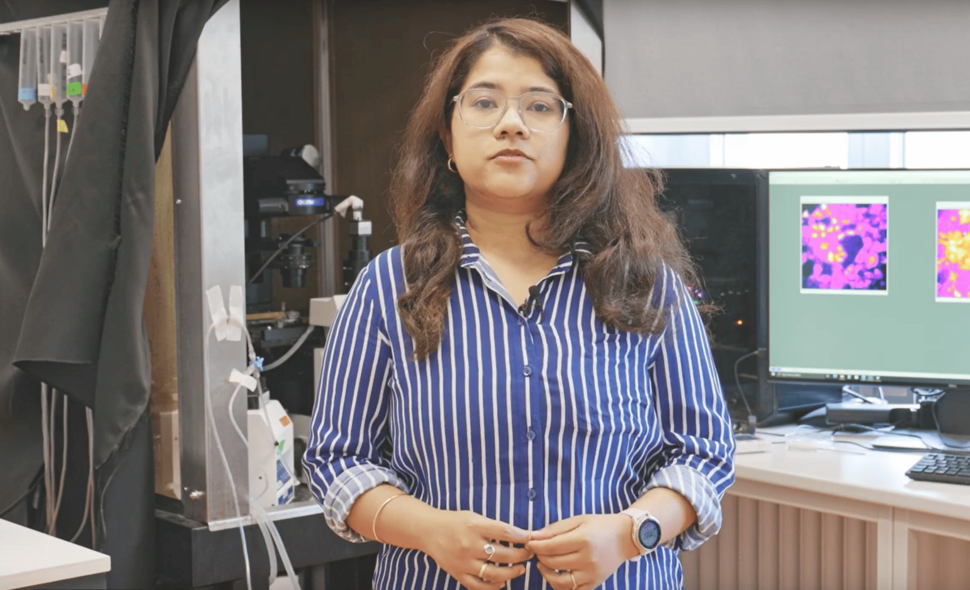
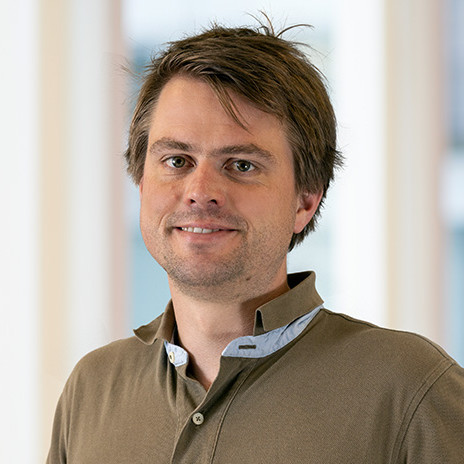
Kontaktinformationen
- Telefon: +49 551 3965526
- Telefax: +49 551 395895
- E-Mail-Adresse: tobias.bruegmann(at)med.uni-goettingen.de
Professor für Physiologie und Pathophysiologie